Quantum Physics in Leaf Photosynthesis: A Natural Phenomenon
Plants utilize quantum superposition in the process of photosynthesis.
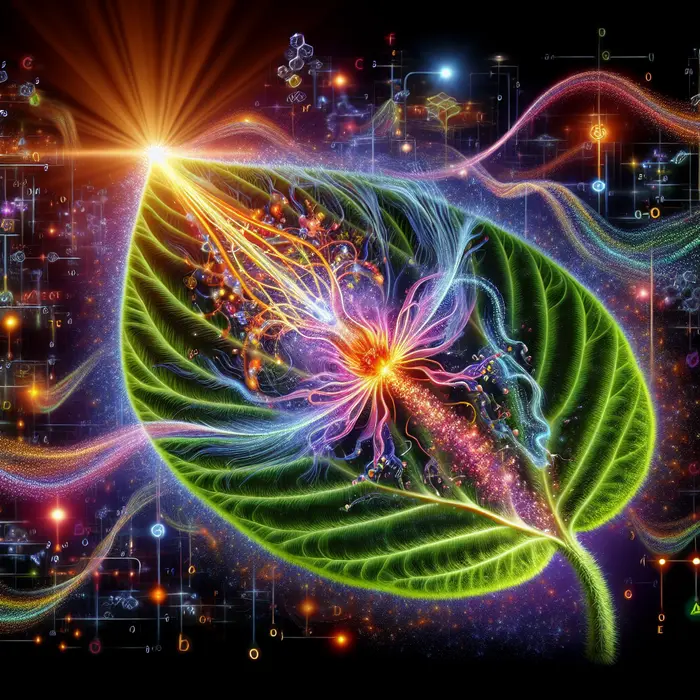
In its traditional sense, physics often appears intuitive when applied to observable phenomena such as the motion of falling objects or the behavior of everyday materials. However, at the quantum level—where the rules governing subatomic particles come into play—physics becomes much less intuitive, frequently defying our common-sense understanding of how the world works. Surprisingly, these mind-bending principles of quantum mechanics are not confined to esoteric laboratory experiments or theoretical physics but play a crucial role in one of the most fundamental biological processes: photosynthesis.
The Process of Photosynthesis
Photosynthesis is when plants, algae, and some bacteria convert light energy into chemical energy. This conversion allows them to fuel their activities and sustain life on Earth. While photosynthesis is essential for the survival of these organisms—and, by extension, all life that depends on oxygen and food—it is far from a perfect system. Remarkably inefficient, plants use only about 1-2% of the sunlight they absorb for energy production. This inefficiency has long puzzled researchers, given the importance of the process.
The Role of Electrons in Photosynthesis
At the heart of photosynthesis lies a series of complex reactions that move electrons through a plant’s photosynthetic apparatus. The goal is to transport an electron from an excited state—triggered by sunlight absorption—to where it can be stored as chemical energy. However, this path is not straightforward; the electron faces multiple decision points along its journey.
Quantum Mechanics in Photosynthesis
Researchers have discovered that rather than choosing a single path to travel, photosynthesis's electrons exhibit quantum superposition behavior. This means that an electron does not take just one route but simultaneously takes multiple paths. Essentially, the electron “samples” every possible route at once to assess the most efficient trajectory.
This phenomenon is further enhanced by quantum coherence, where an electron maintains its superposition across all potential pathways. As a result, it can evaluate which path offers the least resistance and highest efficiency before settling on a final course. This quantum-level optimization allows plants to make better use of absorbed sunlight, compensating for some inherent inefficiencies in photosynthesis.
Implications and Significance
The discovery that quantum mechanics plays a role in photosynthesis has far-reaching implications and unexpectedly bridges biology with physics. It highlights nature’s remarkable ability to incorporate highly complex quantum processes into biological systems previously thought exclusive domains for physicists and specialized technology. By harnessing principles of quantum mechanics, plants maximize their energy efficiency in ways classical physics alone cannot explain.
This intersection between biology and quantum physics underscores nature’s complexity and challenges our understanding of life’s processes. It also provides insight into how quantum mechanics—which typically governs particle behavior at minuscule scales—can have tangible effects on systems as large as plant leaves.
Richard Feynman famously stated: “Nature is not classical dammit, and if you want to make a simulation of nature, you’d better make it quantum mechanical.” The role of quantum mechanics in photosynthesis proves this statement true by demonstrating that even everyday biological processes operate on principles far more complex than we might have imagined.
Conclusion
The involvement of quantum mechanics in photosynthesis deepens our understanding of this vital process and expands our view of how the natural world functions. It suggests that quantum phenomena are not isolated to abstract physics experiments but are woven into life’s fabric on Earth. This revelation opens up exciting possibilities for future research where further study into quantum biology could unlock new ways to enhance energy efficiency—potentially leading to advances in renewable energy and artificial photosynthesis.